Introduction
This blog reviews the current state of research on teaching quantum mechanics in secondary and lower undergraduate education. The conceptual approach to quantum mechanics is being implemented in introductory physics courses worldwide, but research on misconceptions, testing, and teaching strategies for introductory quantum mechanics is needed.
The blog analyzed articles on misconceptions, research tools, teaching strategies, and multimedia applications investigated in teaching quantum mechanics. The outcomes were categorized according to their contribution to various subtopics of quantum mechanics.
The study found that students have difficulty relating quantum physics to physical reality and that the teaching of complex quantum behavior, such as time dependence, superposition, and measurement problems, has barely been investigated for the secondary and lower undergraduate level. At the secondary school level, there is a need to investigate student difficulties concerning wave functions and potential wells.
The study also found a lack of research into which teaching strategies promote understanding and a need for more empirical research into student difficulties, teaching strategies, activities, and research tools intended for a conceptual approach to quantum mechanics. Additionally, there is a need for the development of assessment tools for secondary and lower undergraduate education that cover all major topics and are suitable for statistical analysis.
Uncertainty Principle
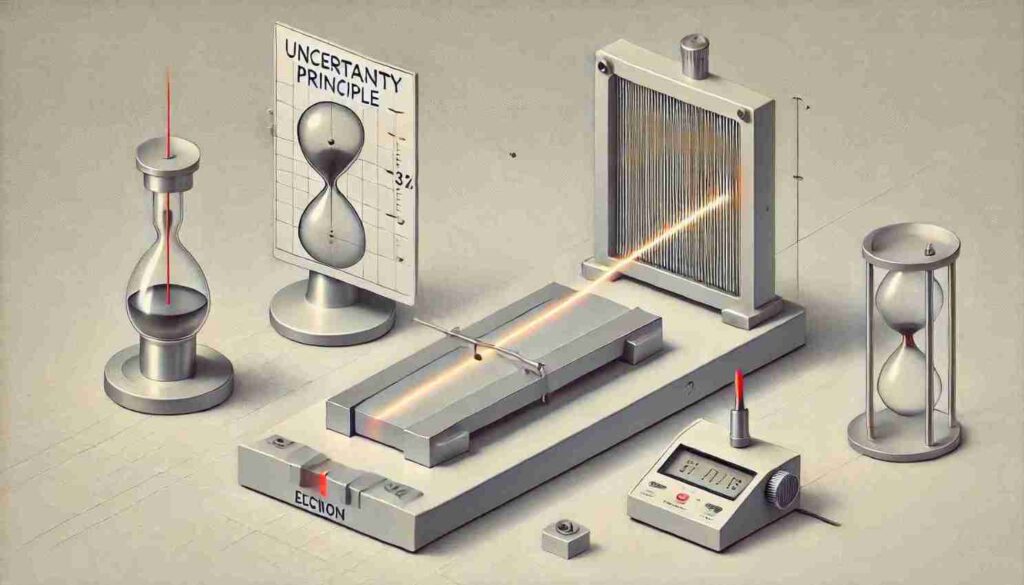
Many students attribute uncertainty to external effects, measurement errors, or instrument errors, rather than recognizing it as an intrinsic property of quantum systems. Some students believe that uncertainty is caused by the high velocity of quantum particles.
CASMIR Effect
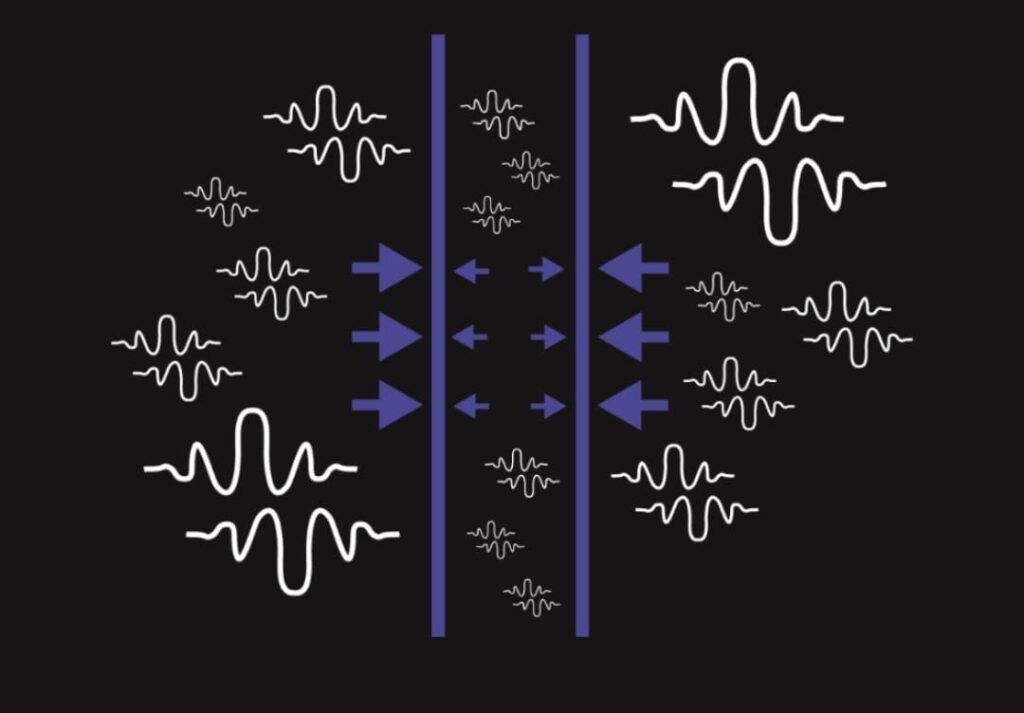
The Casimir effect is a physical phenomenon that proves the existence of virtual particles. It was predicted in 1948 by a Dutch physicist Hendrik Casimir based on the uncertainty principle for time and energy.
Casimir correctly assumed that if we put two parallel uncharged plates just a few nanometres apart, they would attract as a consequence of vacuum fluctuations.
As we have learned in the previous chapter, virtual pairs of particles and antiparticles are being created constantly between and around the plates. However, for a virtual pair to be created between the plates, its wave function must have a relatively small wavelength, since greater wavelengths do not fit between the plates. Consequently, fewer virtual particles are being created between the plates than in other places around the plates, where particles of arbitrary wavelengths can be created. This results in greater pressure on the outside of the plates, and the plates start drawing closer.
Photoelectric Effect
Students often confuse the photoelectric effect with ionization. They struggle to understand how light and electrons interact, and how various aspects (work function, kinetic energy, cutoff frequency, and material properties) constitute the photoelectric effect.
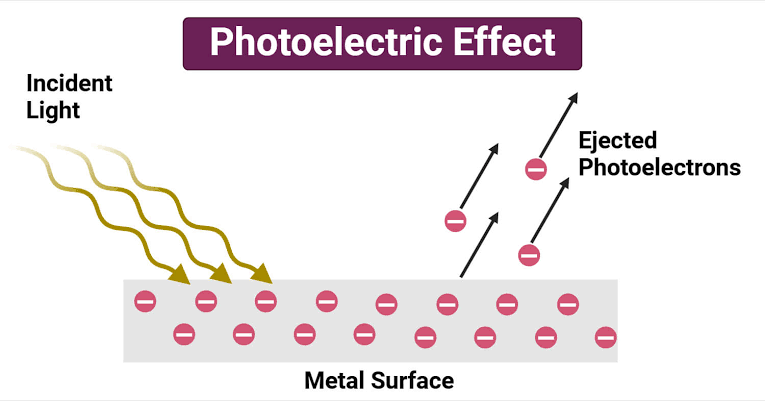
Common misconceptions include:
- Believing that an increase in light intensity will increase the energy transferred to a single electron.
- Thinking that a voltage is needed for the photoelectric effect.
- Falsely attributing the energy transfer to chemical reactions between light and electrons.
Wave Functions
Students often describe wave functions as physical waves, rather than mathematical constructs. They struggle to distinguish between wave functions, energy levels, and probability. Common misconceptions include:
- Believing that wave functions describe particle trajectories.
- Thinking that the amplitude of wave functions measures energy.
- Confusing probability with classical arguments (e.g., velocity).
- Believing that energy is lost during tunneling.
- Falsely attributing the decrease of wave function amplitude to energy loss.
Atomic Structure And Models
Students often hold onto various atomic models, including the planetary model, and struggle to let go of these models. They have difficulty understanding the probabilistic nature of atomic models and the concept of orbitals. Common misconceptions include:
- Believing that atomic models are replicas of reality.
- Confusing orbitals with planetary orbits or concentric shells.
- Falsely attributing spin to an object’s rotation around its axis or core.
Complex Quantum Behavior
Students struggle to understand quantum states, superposition, and time evolution. Common misconceptions include:
- Falsely believing that quantum spin is an object’s rotation around its axis or core.
- Confusing pure superposition with mixed states.
- Believing that the probability of finding a particle in a region must be time-dependent for a time-dependent wave function.
Research Tools For Quantum Mechanics Education
Several research tools have been designed and evaluated to investigate students’ difficulties in understanding quantum mechanics concepts at the secondary and lower undergraduate levels. These tools include:
Multiple-choice concept tests:
Quantum Mechanics Visualization Inventory (QMVI): 25 questions focusing on the interpretation of diagrams, with a focus on conceptual understanding of potential energy and wave functions.
Quantum Mechanics Conceptual Survey (QMCS): 12 questions addressing the conceptual understanding of wave-particle duality, wave functions, potential wells, atomic structure, and quantization.
Quantum Physics Conceptual Survey (QPCS): 25 questions testing conceptual understanding of basic concepts of quantum mechanics, including the photoelectric effect, wave-particle duality, and the uncertainty principle.
Other tools:
Multivariate analysis: a 40-item Likert-scale questionnaire to investigate students’ reasoning and mental models.
Concept map strategy: a tool to evaluate the learning process, diagnose learning difficulties, and map the progression of students’ cognitive structure.
Typology of learning impediments: a framework to analyze underlying causes of students’ difficulties, including lack of prerequisite knowledge, failure to make connections, and inappropriate interpretations.
Questionnaire on atomic structure: a tool to investigate deep understanding and critical thinking of first-year undergraduates regarding the quantum atom model.
Teaching Quantum Mechanics
Research has shown that students tend to hold onto classical models of the atom, such as Bohr’s planetary description, due to their classical worldview. To address this, various approaches have been evaluated, including:
- Emphasizing the differences between classical and quantum mechanics
- Focusing on the historical development of the atomic model
- Using classical analogies to promote understanding of the quantum atom model
- Developing instructional modules that contrast classical and quantum models
- Using the Heisenberg uncertainty principle as a basic principle
These approaches have been shown to improve students’ understanding of quantum concepts, including the hydrogen atom and the Schrödinger model. Additionally, research has explored the relationship between mathematical and conceptual understanding of quantum concepts.
While mathematical skills are necessary for understanding quantum physics, they are not sufficient for conceptual understanding. Non-mathematical approaches, such as focusing on real-life applications and visualization, have been shown to lead to an adequate understanding of quantum concepts.
Active learning strategies, such as peer interaction, interactive engagement methods, and writing activities, have also been found to contribute to a better understanding of quantum concepts. Multimedia applications, including simulations and interactive tutorials, have been developed and evaluated, showing promising results in improving student understanding.
Overall, the research suggests that a combination of approaches, including emphasizing differences between classical and quantum mechanics, using classical analogies, and incorporating active learning strategies and multimedia applications, can help to improve student understanding of quantum mechanics.
Student Difficulties With Quantum Mechanics
Research has shown that students at both secondary and undergraduate levels struggle with understanding quantum mechanics, particularly with complex quantum behavior and wave function.
Many students hold onto classical thinking, leading to a mix-up of classical and quantum concepts. This mix-up is due to an inability to connect quantum behavior to physical reality, resulting in an over-literal interpretation of classical metaphors and the use of classical reasoning in describing quantum processes. Students also have difficulties with wave-particle duality, atomic models, and the use of models in physics.
Research has proposed solutions, such as using well-defined analogies, unambiguous diagrams, and more knowledge of models used in physics. However, the impact of these solutions remains to be investigated.
There is a need for more research into teaching strategies, assessment methods, and the development of more questions to cover all major topics in quantum mechanics. Additionally, more empirical research is needed to investigate the teaching of quantum mechanics at both secondary and undergraduate levels.
Research On Student Understanding Of Quantum Mechanics
Various studies have investigated student understanding of quantum mechanics, using a range of methods including concept cards, interviews, cognitive tests, questionnaires, and observations.
These studies have involved students at different levels, from introductory to advanced, and have explored their understanding of specific concepts such as the atom, wave functions, and quantum tunneling.
The research has identified common difficulties and misconceptions that students have and has developed and tested various instructional materials and approaches to improve student understanding. These include the use of simulations, animations, and multimedia resources, as well as research-based learning tools and tutorials.
The studies have also explored the role of metacognitive beliefs and student characteristics in shaping their understanding of quantum mechanics.