Introduction
The quest to understand the size of a neutrino, a subatomic particle renowned for its elusive nature, embodies a compelling intersection of particle physics and cosmology. For decades, neutrinos have been the subject of intense scrutiny due to their incredibly small mass and weak interaction with matter, leading to significant challenges in measurement and characterization. Despite their abundance, as billions pass through our bodies every second, the question of how big they actually are remains largely unanswered.
Recent advancements in experimental techniques have begun to shed light on this enigmatic particle, facilitating a re-examination of longstanding assumptions in the field. This study not only challenges existing paradigms but also opens avenues for new theories that could potentially revolutionize our understanding of the universe at a fundamental level. Consequently, the ongoing exploration of neutrinos promises to illuminate their physical characteristics, which may ultimately enhance our broader comprehension of particle interactions and the basic structure of matter itself.
Overview of Neutrinos And Their Significance in Particle Physics
Neutrinos are elementary particles that have garnered significant interest within the field of particle physics due to their unique properties and pervasive presence in the universe. These neutral particles, which belong to the lepton family, are produced in various processes, including nuclear reactions within stars and during the decay of radioactive substances.
Despite their abundance outnumbering electrons in the universe by a substantial margin neutrinos interact very weakly with matter, making them incredibly elusive. This elusive nature poses challenges for detection and study, yet it also makes it invaluable for probing fundamental questions about the universe’s structure and evolution. Their ability to oscillate between different flavors, as established by experimental observations, has profound implications for our understanding of mass and the standard model of particle physics.
There are three known types, or “flavors,” of neutrinos: electron neutrinos, muon neutrinos, and tau neutrinos, each associated with their corresponding charged lepton. One of the most surprising discoveries in particle physics was that neutrinos can oscillate, or change from one flavor to another as they travel. This phenomenon, known as neutrino oscillation, was first confirmed in the late 1990s and implies that neutrinos must have mass contrary to earlier assumptions in the Standard Model of particle physics.
The discovery of neutrino oscillation led to a Nobel Prize in Physics in 2015 and opened up new avenues in the study of fundamental physics. As research continues to unveil the complexities of neutrinos, scientists are gradually piecing together their intricate role in the cosmos and addressing the basic question of their size and significance.
Like to Read: Unlocking the Secrets of Molecular Structures: A Breakthrough Discovery
The Historical Context of Neutrino Research
The journey of neutrino research is deeply rooted in the early developments of particle physics, which began in the 1930s with Wolfgang Pauli’s theoretical hypothesis. In 1930, Pauli proposed the existence of a yet-undetected particle to provide a solution to the conservation of energy in beta decay processes. This groundbreaking idea was further solidified by Enrico Fermi in 1934, who incorporated the neutrino into his theory of weak interactions, providing a framework that enhanced understanding of subatomic processes.
However, it wasn’t until 1956 that experimental evidence for the neutrino’s existence was confirmed by Clyde Cowan and Frederick Reines, who detected these elusive particles generated by a nearby nuclear reactor. This pivotal moment marked the beginning of a dedicated field of study, leading to a series of experiments that increasingly refined our understanding of neutrino’s properties, such as their incredibly small mass and lack of electric charge, laying the groundwork for contemporary research that continues to unveil their mysteries.
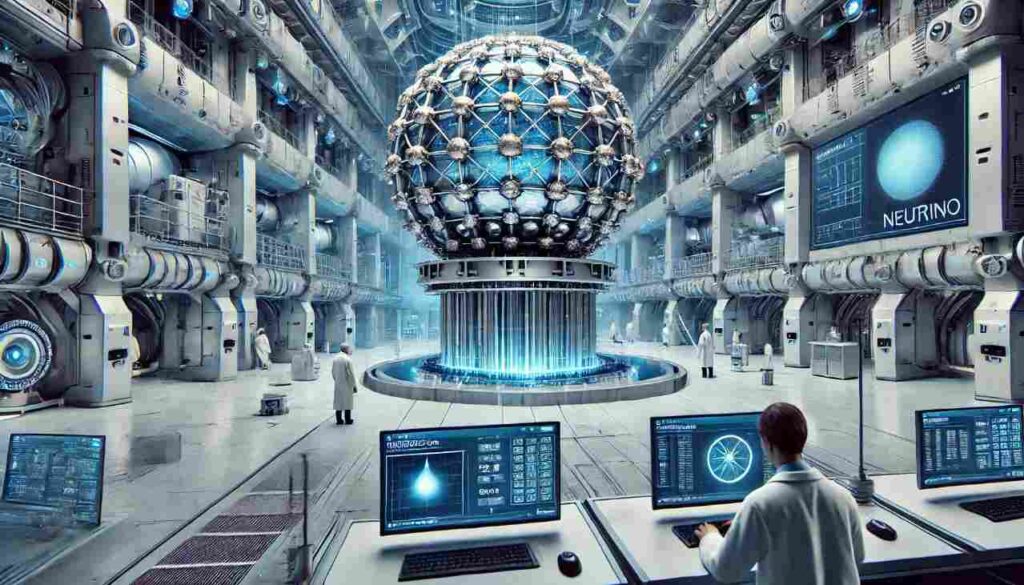
Key Experiments And Discoveries That Shaped Our Understanding of Neutrinos
The exploration of neutrinos has undergone significant evolution, driven by pivotal experiments that have refined our understanding of these elusive particles. Initially, neutrinos were hypothesized by Wolfgang Pauli in 1930 to account for missing energy in beta decay, a concept later substantiated by the detection efforts led by Clyde Cowan and Frederick Reines in the 1950s, who successfully confirmed the existence of neutrinos through their landmark experiment utilizing antineutrinos emitted from a nuclear reactor.
Following this breakthrough, the development of large detectors, such as the Super-Kamiokande in Japan, further advanced the study of neutrinos, unveiling phenomena like neutrino oscillation, which revealed that neutrinos have mass an insight that challenged prior notions of particle physics. Additionally, observations from astrophysical sources, including the Sun and supernovae, have illuminated the varied behaviors and characteristics of neutrinos, collectively painting a more nuanced portrait of their role in the universe and promoting ongoing discussions about their fundamental nature.
Also Read: Scientists Make History with Atom Bending
Recent Advances in Neutrino Measurement
Recent advances in neutrino measurement technology have significantly enhanced our understanding of these elusive particles, enabling physicists to probe their properties with unprecedented precision. Innovations such as highly sensitive photodetectors and advanced liquid scintillator detectors have improved the detection rates of neutrino interactions, allowing researchers to collect more substantial and coherent data. For example, experiments utilizing large-scale neutrino observatories, such as the Deep Underground Neutrino Experiment (DUNE)and the Super-Kamiokande facility, have shifted conventional paradigms by exploring oscillation patterns that reveal important information about neutrino masses.
Furthermore, the integration of artificial intelligence and machine learning techniques into data analysis processes has streamlined the identification of neutrino signals in backgrounds cluttered with noise, yielding more accurate measurements. Collectively, these advancements not only provide critical insights into the size and mass of neutrinos but also open new avenues for understanding their role in the fundamental forces of the universe. This breakthrough illuminates the complex interplay between subatomic particles and the fabric of reality itself.
Innovative Techniques and Technologies Used to Determine Neutrino Size
Recent advancements in experimental physics have introduced innovative techniques and technologies that enhance our understanding of the elusive neutrino, particularly in determining its size. One promising approach utilizes high-precision detectors equipped with advanced photon sensors, enabling researchers to capture faint signals generated by neutrinos interacting with matter. These detectors, often situated deep underground or within the ocean, minimize background noise and allow for fine measurements of neutrino interactions.
Additionally, novel computational algorithms, leveraging machine learning and artificial intelligence, have transformed how data from these experiments is analyzed, offering unprecedented clarity and efficiency. By simulating potential interaction outcomes, scientists can better infer the properties of neutrinos, including their size. Furthermore, cutting-edge collaborations, such as those seen in large-scale international projects, have brought together diverse expertise and resources, propelling neutrino research into a new era. As these techniques mature, they promise to provide increasingly accurate assessments of the neutrino’s characteristics, bridging significant gaps in our current understanding
Conclusion
Despite being incredibly small and nearly massless, they are one of the most abundant particles in the cosmos. Their weak interactions with matter make them difficult to detect, yet they provide invaluable insights into processes such as nuclear fusion in stars, supernovae, and the inner workings of the Sun. The ongoing investigations into the size of neutrinos mark a significant advancement in our understanding of fundamental particles and the intricate framework of the universe.
As sophisticated experiments are developed, scientists are beginning to piece together the elusive nature of neutrinos, revealing that these minuscule particles may have mass, albeit extremely small. These discoveries challenge long-held assumptions and urge a reevaluation of theoretical models that describe particle interactions and the nature of matter itself. Moreover, the implications of defining the neutrino’s size extend beyond particle physics; they resonate within cosmology and our comprehension of the universe’s evolution. As researchers continue to refine their methodologies and uncover deeper insights, the journey to comprehensively understand neutrinos serves as a reminder of the dynamic nature of scientific inquiry.
Ultimately, the answers we seek regarding neutrinos’ size may unlock keys to greater mysteries, bridging gaps in our knowledge and expanding the horizons of modern physics. Ongoing research into neutrino properties, such as their mass and oscillation behavior, continues to challenge and refine the Standard Model of particle physics, highlighting the importance of neutrinos in both astrophysics and particle physics.
Incredible perspective! Your thoughts on digital creativity align perfectly with Sprunki‘s innovative approach. The way Sprunki combines accessibility with depth creates an unmatched musical experience. It’s a game that touches the heart!
Wow! Thank you! I constantly wanted to write on my blog something like that. Can I implement a portion of your post to my blog?
sure.